Nuclear Meltdown Relocation and Core Catcher Analysis
DOI:
https://doi.org/10.14295/vetor.v33i2.15158Keywords:
Nuclear reactor core, Meltdown relocation, Core Catcher, Lower plenumAbstract
Nuclear meltdown with the potential human and environmental harm is one of the major accident hazard (MAH) faced by nuclear power plants. Limiting (or entirely avoiding) criticality events are the main design strategies for reactors of generations 3½ and 4 (Gen3½ and Gen4). These include ensuring negative void and negative temperature coefficients (for both moderator and fuel) regardless of operational conditions, which provide a self-regulating mechanism that helps preventing accidents occurrence (i.e., to address safety and reliability aspects of Gen4’s goals). However, in severe accident scenarios (e.g. during loss-of-coolant, LOCA, events) where failure to extract heat from the reactor may lead to core degradation, strategies to mitigate reactor meltdown and relocation are critical in the design of safety protocols. This work aims to numerically investigate core relocation as an integrated multi-fluid and heat dynamics problem in which flow of melted materials (UO2, Zircaloy and graphite) are modelled through interface capturing/tracking methods. Two interface tracking/capturing methods were compared, the level-set volume of fluid method (VOF) in Ansys Fluent, and the compressive advection method (CAM) in Fluidity/ICFERST. Both methods are in good agreement for the core relocation simulation. An in-vessel core catcher (IVCC) of tungsten alloy was also proposed to demonstrate core degradation control strategy through cooling of the melted multi-materials. The IVCC was simulated with a multifluid model in Ansys Fluent, in a specified applied heat flux model. The thickness of the IVCC is 0.20 m and the heat flux used is 600 kW m-2. The tungsten material used was able to withstand both thermal and mechanical loads on the lower plenum by extracting decay heat from the corium.
Downloads
References
H. Madokoro and I. Sato, “Estimation of the core degradation and relocation at the fukushima daiichi nuclear power station unit 2 based on RELAP/SCDAPSIM analysis,” Nuclear Engineering and Design, vol. 376, p. 111123, 2021. Available at: https://doi.org/10.1016/j.nucengdes.2021.111123
B. Sehgal, Nuclear Safety in Light Water Reactors: Severe Accident Phenomenology. Elsevier Science & Technology Books, 2012. Available at: https://books.google.co.uk/books?id=Z_SmDAEACAAJ
T. Feng, W. Tian, P. Song, J. Wang, M. Wang, L. Li, G. Su, and S. Qiu, “Spatial temperature distribution of fuel assembly pre-simulation for a new simple core degradation experiment,” Progress in Nuclear Energy, vol. 111, pp. 174–182, 2019. Available at: https://doi.org/10.1016/j.pnucene.2018.11.004
A. Nayak, P. Kulkarni, P. Pandey, and S. V. Prasad, Advances of Computational Fluid Dynamics in Nuclear Reactor Design and Safety Assessment. Woodhead Publisher, 2019, ch. Modelling of core melt scenarios in nuclear reactors, pp. 487–565.
J. Gomes, C. Pain, M. Eaton, A. Goddard, M. Piggott, A. Ziver, C. de Oliveira, and Y. Yamane, “Investigation of nuclear criticality within a powder using coupled neutronics and thermofluids,” Annals of Nuclear Energy, vol. 35, no. 11, pp. 2073–2092, 2008. Available at: https://doi.org/10.1016/j.anucene.2008.05.012
ANSYS, ANSYS Fluent Theory Guide 2021R1, ANSYS, 2021.
D. Pavlidis, J. Gomes, Z. Xie, J. Percival, C. Pain, and O. Matar, “Compressive advection and multi-component methods for interface-capturing,” International Journal for Numerical Methods in Fluids, vol. 80, no. 4, pp. 256–282, 2016. Available at: https://doi.org/10.1002/fld.4078
G. H. Yeoh and T. Barber, “Assessment of interface capturing methods in computational fluid dynamics (CFD) codes — a case study,” The Journal of Computational Multiphase Flows, vol. 1, no. 2, pp. 201–215, 2009. Available at: https://doi.org/10.1260/175748209789563946
N. D. Katopodes, “Chapter 13 - level set method,” in Free-Surface Flow, N. D. Katopodes, Ed. Butterworth-Heinemann, 2019, pp. 804–828. Available at: https://doi.org/10.1016/B978-0-12-815485-4.00019-X
A. N. Brooks and T. J. Hughes, “Streamline upwind/petrov-galerkin formulations for convection dominated flows with particular emphasis on the incompressible navier-stokes equations,” Computer Methods in Applied Mechanics and Engineering, vol. 32, no. 1, pp. 199–259, 1982. Available at: https://doi.org/10.1016/0045-7825(82)90071-8
J. Crank and P. Nicolson, “A practical method for numerical evaluation of solutions of partial differential equations of the heat-conduction type,” Mathematical Proceedings of the Cambridge Philosophical Society, vol. 43, pp. 50–67, 1947. Available at: https://doi.org/10.1017/S0305004100023197
D. Pavlidis, J. Gomes, Z. Xie, C. Pain, A. Tehrani, M. Moatamedi, P. Smith, A. Jones, and O. Matar, “Numerical modelling of melt behaviour in the lower vessel head of a nuclear reactor,” Procedia IUTAM, vol. 15, pp. 72–77, 2015, iUTAM Symposium on Multiphase Flows with Phase Change: Challenges and Opportunities. Available at: https://doi.org/10.1016/j.piutam.2015.04.011
Thermophysical Properties of Materials for Nuclear Engineering: A Tutorial and Collection of Data, ser. Non-serial Publications. Vienna: INTERNATIONAL ATOMIC ENERGY AGENCY, 2009. Available at: https://www.iaea.org/publications/7965/thermophysical-properties-of-materials-for-nuclear-engineering-a-tutorial-and-collection-of-data
W. Ma, Y. Yuan, and B. R. Sehgal, “In-vessel melt retention of pressurized water reactors: Historical review and future research needs,” Engineering, vol. 2, no. 1, pp. 103–111, 2016. Available at: https://doi.org/10.1016/J.ENG.2016.01.019
P. Tolias, “Analytical expressions for thermophysical properties of solid and liquid tungsten relevant for fusion applications,” Nuclear Materials and Energy, vol. 13, pp. 42–57, 2017. Available at: https://doi.org/10.1016/j.nme.2017.08.002
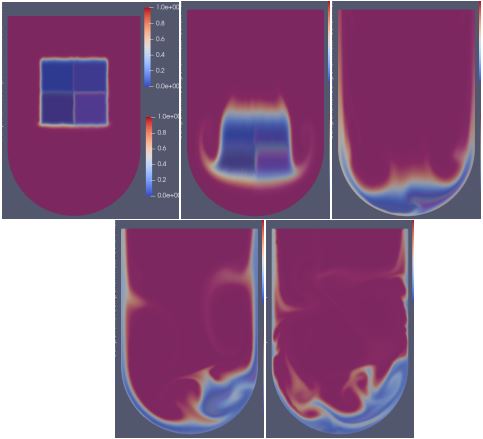